LAS News
Read article:Understanding the future through the land around us
Read article:Understanding the future through the land around us
›
Understanding the future through the land around us
Chunyuan Diao develops better ways to examine ecosystems
Read article:Wendy Heller named Marjorie Roberts Professor in Liberal Arts and Sciences
Read article:Wendy Heller named Marjorie Roberts Professor in Liberal Arts and Sciences
›
Wendy Heller named Marjorie Roberts Professor in Liberal Arts and Sciences
A lifelong pursuit of psychology began with a bicycle wreck
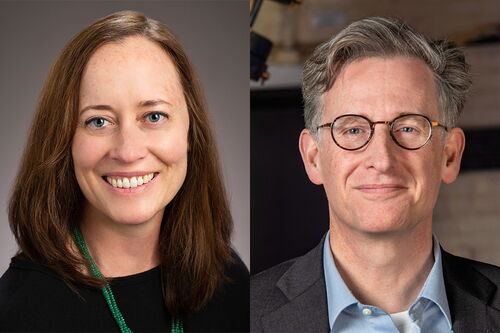
Read article:
Read article: ›
Two LAS faculty elected to American Academy of Arts and Sciences
Bell, Gammie honored for excellence in biological and physical sciences
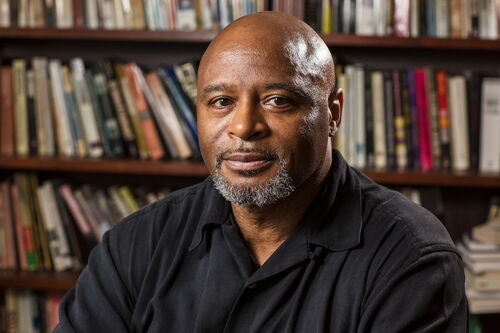
Read article:
Read article: ›
Why is the reparations movement gaining momentum in the U.S.?
Professor: Trend coincides with a retreat from racial justice
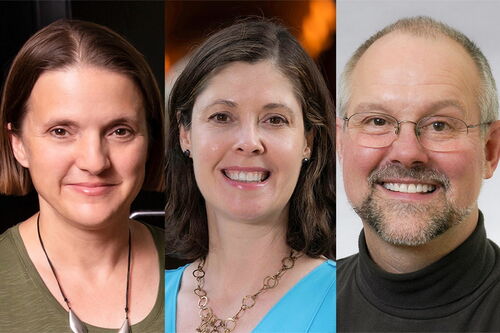
Read article:
Read article: ›
Three LAS professors elected AAAS Fellows
Faculty members honored by world's largest general scientific society
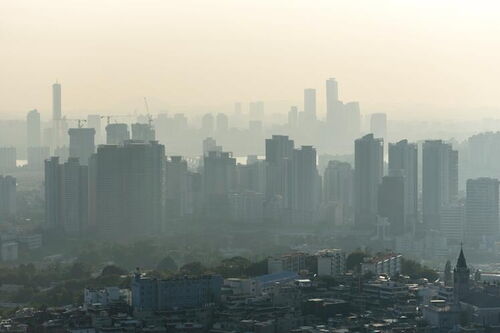
Read article:
Read article: ›
Study identifies atmospheric and economic drivers of global air pollution
Analysis links emissions to demand and consumption among countries
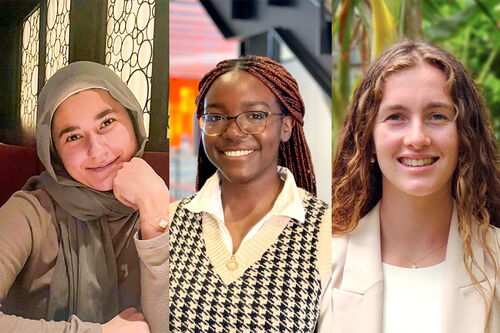
Read article:
Read article: ›
Three LAS students receive Critical Language Scholarships
Department of State program enables 8-10 weeks of studying abroad
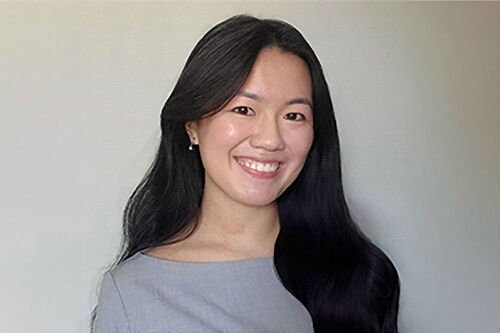
Read article:
Read article: ›
College of LAS student honored with Goldwater scholarship
Sylvia E, a junior, plans to study early development
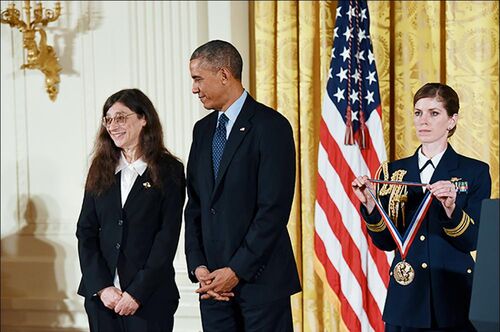
Faculty honors
LAS professors bring honor and recognition to the college. See a list of their accomplishments.
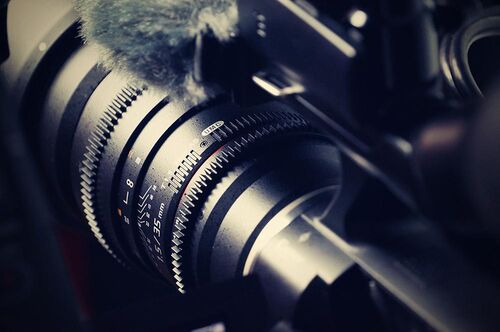
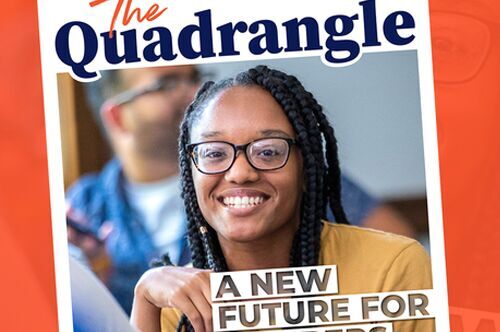
Read The Quadrangle and LAS News
The College of Liberal Arts & Sciences mails its magazine, The Quadrangle, twice a year. Its enewsletter, LAS News, mails monthly.